Reinforced Plastics Move into Non-Traditional Markets
Composites improve performance, reduce mass and costs, increase design versatility, and extend use life
Previous Article Next Article
By Peggy Malnati
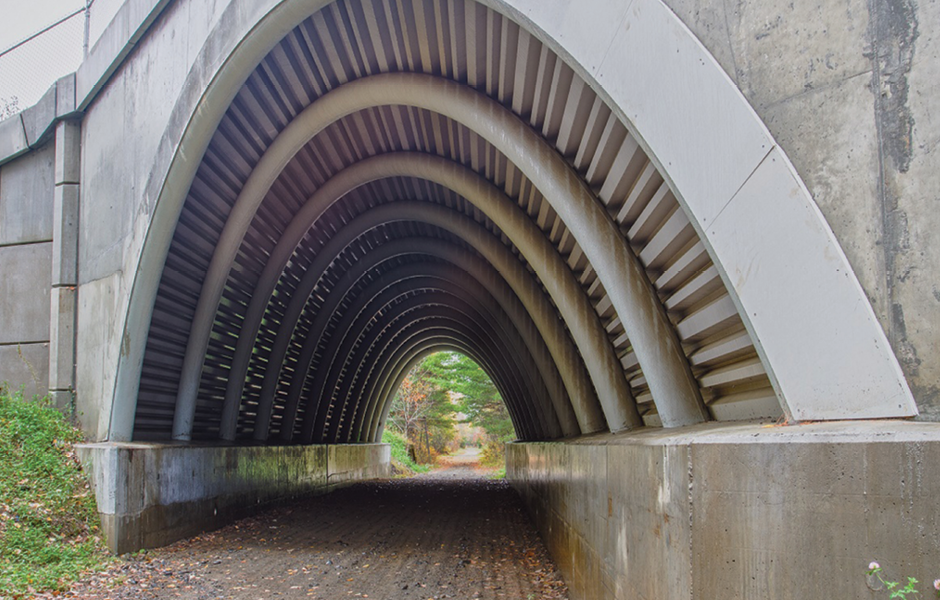
Composites are helping speed up bridge construction (see the second section of this article).
Composites have a long history of use in industries such as automotive, aviation/aerospace, and sporting goods, but their use is now expanding into less-traditional markets like medical, infrastructure, power transmission, and building & construction, where they are displacing metals, wood and wood products, and even concrete. Composites are moving into non-traditional segments for a variety of reasons: they improve part performance, reduce mass and costs, increase design flexibility through parts consolidation, reduce or eliminate secondary operations, don’t corrode or rot, extend durability and use life, increase production speeds, and produce more consistent products. Some interesting examples of recent applications in non-traditional markets are below.
Hyperbaric Oxygen Therapy Chambers
Hyperbaric (above atmospheric pressure) oxygen therapy (HBOT) is best known for saving divers with “the bends” (decompression sickness), but it’s also a well-documented medical treatment for many chronic and acute conditions (i.e., altitude sickness, severe anemia, brain abscesses, carbon-monoxide poisoning, arterial embolism, radiation injuries, and sudden vision or hearing loss). Because HBOT temporarily enables blood to carry more oxygen, it also helps fight infection, reduces inflammation, and speeds healing in cases of crushing injuries, skin/bone infections, skin grafts, and burns.
Traditionally HBOT sessions were administered in rigid single- or multi-person chambers capable of pressures up to 3 ATA (atmosphere absolute, i.e., pressure above normal atmospheric pressure). These heavy glass and metal units were permanent installations that were costly to install and maintain, so treatment costs were high and chambers were only available at large hospitals or naval/diving and recompression centers.
More recently, single-person, soft-sided portable systems capable of pressures to 1.4 ATA were introduced. These polymer-coated fabric chambers don’t provide the same efficacy as the higher-pressure rigid systems, but they are less costly to purchase and maintain, making them affordable for small clinics and individuals. They also weigh far less so are portable/transportable and can be stowed until needed.
Now, a new composites-intensive hyperbaric chamber bridges the gap between costly/high-pressure units and portable units operating at less than half the pressures. The HematoCaresystemfrom Groupe Médical Gaumond (GMG; Montréal, Quebec, Canada) took a decade to develop, and later this year will become the first HBOT system to achieve global agency approvals. Working with National Research Council Canada’s Industrial Research Assistance Program (IRAP; Ottawa, Ontario, Canada), Cytec Industries Inc. (Woodland Park, New Jersey, USA), and Composites Atlantic Ltd. (Lunenburg, Nova Scotia, Canada), the team’s goal was to develop a lightweight chamber capable of maintaining 3 ATA without leaking or tearing, but still affordable for small clinics and individuals. (Certification at 3 ATA operating pressures means chambers are tested at pressures six-times higher, so mechanical requirements are demanding and flame-retardancy standards stringent, owing to oxygen’s combustibility.)
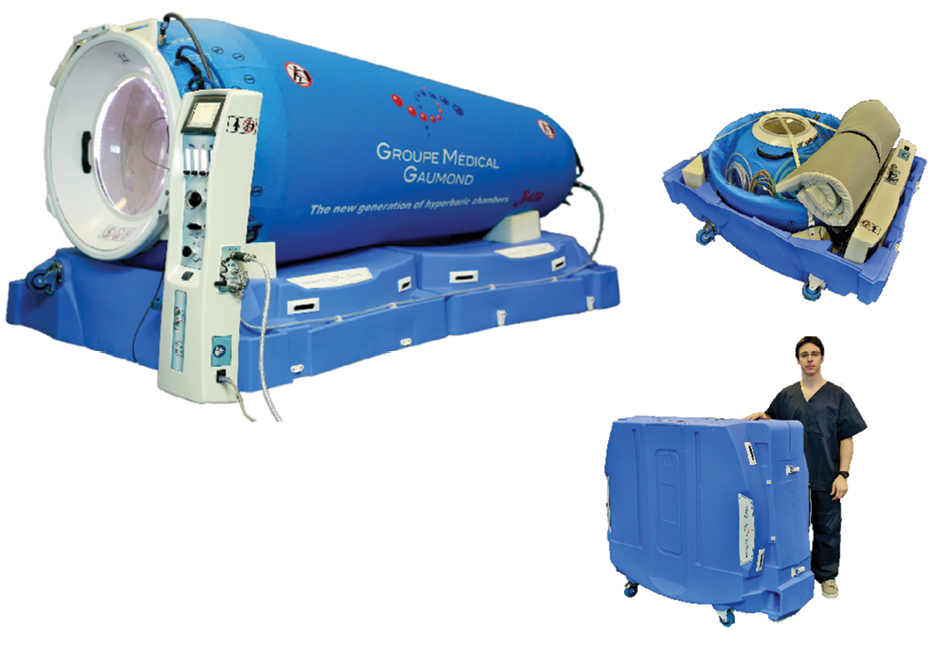
Hyperbaric oxygen therapy (HBOT) is a medical treatment for a variety of chronic and acute conditions. A new type of composites-intensive hyperbaric chamber has a cone-shaped hollow chamber that’s filament wound using high-performance aramid fiber impregnated with urethane. Rigid composite end caps (infused with gel-coated, fiberglass-reinforced epoxy and fitted with curved acrylic windows) are held snuggly in place on either end of the flexible chamber by pressure alone and provide patient egress. All standard system components pack into a tough two-piece HDPE rotomolded case with casters (insets). (Photos courtesy of Groupe Médical Gaumond.)
Years of testing and evaluation led to development of a filament-wound cone-shaped hollow chamber produced by Composites Atlantic using high-performance aramid fiber from Teijin Ltd. (Osaka, Japan) impregnated with custom-formulated urethane.
Rather than make ill patients enter through a double-zippered opening at the top, like with most soft-sided units, GMG eases patient access by creating door- and window-equipped rigid composite end caps that fit snuggly into the flexible chamber yet aren’t permanently attached. GMG infuses the parts in gel-coated, fiberglass-reinforced epoxy. After post-mold trimming, an aluminum support ring is bonded into each end cap followed by insertion of a rubber O-ring, a curved acrylic window, and a final aluminum locking ring. Clever design inside the end caps allows optional rails to be added to support patients entering on stretchers.
All standard system components pack into a tough, two-piece case with casters that’s rotomolded from unreinforced high-density polyethylene. When the chamber is deployed, carrying-case halves open to form a base that supports the chamber, while foam blocks prevent it from rolling. With experience, two people can deploy the system in 15 minutes and repack it in half that time. The system is warranted for ten years/4,000 cycles and, with a 125-kg packed weight, is said to be the lightest, least-expensive HBOT chamber certified to 3 ATA working pressures, making it adaptable for home, clinic, or hospital use.
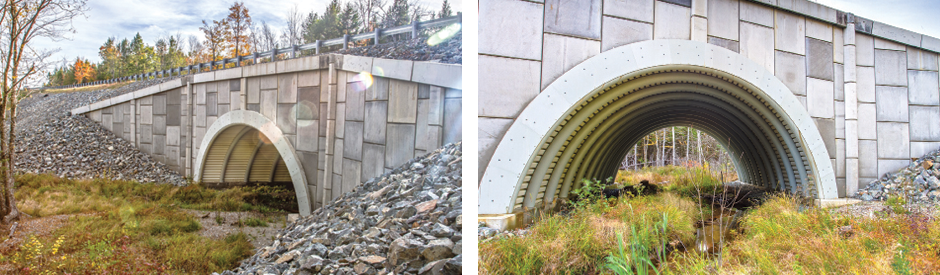
A composites-intensive bridge technology developed by the University of Maine is said to provide a better and faster way to replace a wide variety of concrete and other bridges. The composite bridge uses “inflatable arches”―Portland cement-filled resin-infused textile sleeves that provide a permanent form for concrete—creating an exoskeleton that eliminates the need for rebar and protects concrete from water and environmental damage. (Photos courtesy of University of Maine.)
Composites for Rapid Bridge Replacement
With increasingly unpredictable weather, natural disasters, and civil unrest plaguing many regions, it’s increasingly important to be able to replace damaged or destroyed bridges rapidly. Even in settled areas, aging infrastructure on bridges that are over their limit and beyond their service life means local and regional governments need ways to replace bridges quickly and cost effectively, preferably with materials offering longer use life.
Traditional precast and cast-in-place concrete bridges have issues. Precast components are very heavy and costly to move, while extensive formwork must be built before casting-in-place, followed by long waits as the concrete cures, adding weeks or months to jobs, particularly in cold and wet conditions. Although fully cured concrete is durable—withstanding broad temperature fluctuations, high winds, heavy loads, and abrasion—it can be affected by cold-weather salt deicers used on roads and bridges (causing concrete spalling and rebar corrosion) and water intrusion (necessitating installation and maintenance of expansion joints to prevent freezing water from splitting components). Both situations eventually can compromise bridge integrity.
Additionally, concrete’s high specific gravity adds significant “dead weight,” reducing a bridge’s true carrying capacity. The shear mass of precast components and the high labor requirements of cast concrete require the use of heavy equipment and large work crews. This increase risks for negative environmental impacts and often requires environmental-impact studies be conducted prior to permitting, slowing projects and adding cost. Furthermore, shutting down busy bridges for repair/rebuilding increases traffic delays and commute times, frustrates commuters, and can harm local commerce, all of which mean municipalities want bridges fixed and back in service as soon as possible.
A composites-intensive bridge technology developed by University of Maine’s Advanced Structures & Composites Center and commercialized by Advanced Infrastructure Technologies (both in Orono, Maine, USA) is said to provide a better, faster way to replace a wide variety of concrete bridges, freeway underpasses/overpasses, and railroad bridges. The project began with three ambitious goals: replace concrete formwork and rebar, use efficient arched structures, and produce components at the worksite.
Researchers reviewed and rejected many design options before deciding to produce formworks using inflatable fabric sleeves. Ultimately a proprietary fabric blending glass, carbon, and other fibers and infused with epoxy-vinyl ester resin (selected for its durability, ductility, and favorable adhesion to concrete) was developed. In use, the custom-designed sleeves are cut to length and width, joined to form a tube, and mounted to custom fixtures. Tube ends are then sealed and sleeves are inflated with air prior to resin impregnation. Cure occurs at room temperature and normal pressures.

A pultruded 3-D composite technology called Transonite developed by Ebert Composites Corp. is used for a wide variety of lightweight panels, providing reinforcement in all three orthogonal directions. A variety of fibers, fabrics, and thermoset or thermoplastic resins can be used, making it easy to tailor performance, cost, and mass to meet application needs. An internal “wave” pattern of reinforcement is said to withstand deflection and high shear forces. (Photo courtesy of Creative Pultrusions, Inc.)
Arches are then moved to a job site, positioned, and concrete footings are poured. Next, holes are cut at the top of each arch and a proprietary grade of Portland cement (which is slightly expansive and bonds well to the composite sleeve) is poured into each arch and allowed to cure. Then decking (conventional or composite) is installed over the arches, sand backfill is used, and a final wear surface (asphalt or polymer concrete) is applied.
Functionally, inflatable arches provide a permanent form for concrete, create an exoskeleton that eliminates the need for rebar, and protect concrete from water intrusion and other environmental damage. Installed costs can be 20% lower than conventional materials, yet these joint- and rebar-free bridges are designed to last at least twice the typical 50- to 70-year lifespan of conventional bridges. Eliminating expansion joints and rebar means the new technology is less prone to fatigue, corrosion, and water infiltration, reducing maintenance costs and time. Smart sensors can be installed for active bridge monitoring. A small bridge can be completely replaced in as little as one week, versus months with conventional construction.
Durable 3-D Composite Panel Structures
A unique pultruded 3-D composite developed by Ebert Composites Corp. (Chula Vista, Califorinia, USA) and licensed by Creative Pultrusions, Inc. (Alum Bank, Pennsylvania, USA) provides x, y, and z-axis reinforcement (structural fibers in all three orthogonal directions). This increases panel durability by greatly improving peel strength and delamination resistance and boosting torsional rigidity. Other benefits include extremely high strength/weight ratios, thermal and acoustical insulation, and elimination of corrosion.
Called Transonite, the technology (now in its third generation) is covered under nine U.S. patents and is produced on unique equipment via an automated process (both developed by Ebert) capable of supporting high-volume manufacturing at low costs. Functioning like I-beams, z-axis fibers are precut rovings (e.g., glass, carbon, aramid, basalt) that are pushed through top and bottom skins with or without a core in place. The “clinched/riveted” rovings in one discrete fiber bundle aren’t connected to fibers in adjacent bundles (fibers are not stitched together), yet the unbroken fibers form regular “columns” in the through-thickness direction. Cores may be solid or foamed resins or even balsa wood.
Special tooling keeps skins and fiber columns from collapsing prior to infusion/impregnation, which ties fibers, cores, and skins. Additional skin layers are added to lock rovings into original skins and improve surface finish. Finally, the pultruded sandwich is pulled through a heated die to catalyze and cure the resin, which typically is a thermoset (unsaturated polyester, vinyl ester, or polyurethane) but also can be thermoplastic.
Thanks to process versatility, a wide variety of fibers, fabrics, and resins can be used, making it easy to tailor performance, cost, and mass to meet application needs. Performance can be changed by manipulating panel thickness (13-102 mm), skin thickness (1.27-10.2 mm), skin/core ratio, panel width (15-259 cm), and density of z-axis fibers (0.08-2.48/cm2). Since it’s a continuous process, panels can be cut to nearly any length greater than 2.5 cm, yielding large, clean surfaces free of seams, which is important in situations where moisture intrusion is a concern and/or aesthetics are important.
The angle of z-axis fiber placement is another interesting variable. While 90° degrees to the skins is most common, recent developments permit addition of very-high-shear layers of fibers positioned nearly 45° to the z-axis prior to resin impregnation, creating an internal “wave” pattern that withstands deflection and high shear forces.
Post-mold trimming is done via waterjet or diamond tools. Bare panels can be finished with a variety of surfaces for interior or exterior use, including carpet, antiskid aggregate, wallpaper, and polyurea.
Transonite technology is currently used for floors and sidewalls on recreational vehicles and truck trailers and was demonstrated on a small building in a Chinese solar competition. Other uses include panels for ballistic protection, air-cargo containers, marine uses, furniture, rails, bridge decks, industrial mats, and automotive uses.
Composites for Power Transmission
In an industry long dominated by metals, uses of composites in high-voltage overhead electrical conductors are increasing power-transmission capabilities and capacities. As populations increase and nations consume more power, much of the planet’s electrical-transmission infrastructure is outdated and exceeding its capacity limits. Load growth aside, utilities in many countries legally are required to connect to increasing amounts of solar and wind energy generated by public and private sources, which expands and further stresses aging electrical infrastructure.
To meet projected demand and accommodate alternative power generation, electric utilities have three ways to increase system capacity:
- build new lines (a costly approach requiring difficult-to-secure public consent and rights-of-way for land, and even longer waits for permitting and construction);
- “reconductoring” (restringing) existing lines with conductor of the same type (which may necessitate strengthening or replacing existing towers and poles, and still involves waits for permitting and construction); or
- reconductoring existing lines with more efficient conductor (the fastest and least costly approach).
Overhead transmission lines must meet many challenging standards, including minimum clearance to ground, ice and wind loads, and thermal-operating limits to prevent damage. Lines are designed to maintain a safe distance from the ground at a rated electrical load under specific environmental conditions. Line sag and therefore ground clearance are affected by both thermal and mechanical issues.
When lines are strung over areas with mountains, bodies of water, or prone to severe weather, higher performance high-temperature low-sag (HTLS) conductor is typically required instead of conventional aluminum conductor steel-reinforced (ACSR) or other alloy-based products. Conductors using lighter, stronger core materials with broader thermal performance can transmit more power during both normal and contingency operations while maintaining required line clearance.
Recent developments in high-strength, low-sag lightweight composite cores to replace stranded steel cores have changed how transmission lines are designed. Carbon fiber-reinforced epoxy cores typically use a single (monolithic) rod rather than multiple strands traditionally used with steel cores to eliminate a single point of failure. Monolithic cores require a larger bending radius than traditional stranded cores and must be handled carefully by trained technicians using special connectors and terminations.
Another composite product features aluminum metal-matrix composites (MMCs, aluminum oxide fibers in a high-purity aluminum matrix). The multi-strand MMC products use traditional fittings but also have a higher bend radius than traditional ACSR conductor. They are rated for high operating temperatures (to 240°C). However, their high cost means they are typically used for only the most restrictive installation environments.
Given the limitations of current HTLS composite conductors, Southwire Company, LLC (Carrollton, Georgia, USA, one of the world’s largest wire and cable producers), and Celanese Corp. (Dallas, Texas, USA) have jointly developed a new type of HTLS product called C7™ Overhead Conductor. The multi-strand pultruded thermoplastic-composite core combines carbon fiber-reinforced polyphenylene sulfide (PPS) with a polyetheretherketone (PEEK) capping layer for very-high resistance to chemicals, heat, galvanic corrosion, and abrasion.
The new conductor carries approximately twice the current as conventional ASCR conductor and provides a high continuous-use (180°C) and even higher emergency-use (225°C) rating, helping utilities maximize energy throughput. The installation-friendly conductor provides greater flexibility and bending radius than monolithic thermoset and MMC composites and works with conventional fittings, reducing crew training time and increasing familiarity. A multi-strand core means greater reliability and redundancy during installation and long-term use compared with monolithic-core conductor.
The new product combines high performance, excellent value, and the toughness and durability to deliver at least a 40-year service-life expectancy, helping utilities increase capacity without the cost of installing new infrastructure. By reducing power losses, transmission is more efficient, and utilities deliver more billable power to customers while avoiding infrastructure upgrades, which also benefit power consumers and reduce environmental impact of the power-transmission grid.
Composite Foundation Walls for Homes
A new composite-based foundation-wall system introduced last year by Composite Panel Systems, LLC (Eagle River, Wisconsin, USA) with support from panel fabricator Fiber-Tech Industries, Inc. (Spokane, Washington, USA) and resin supplier Ashland Performance Materials (a commercial unit of Ashland Inc., Dublin, Ohio, USA) is set to overturn the century-old trend of using poured concrete to form vertical foundation walls on residential homes, at comparable costs, faster installation, and far-better performance.
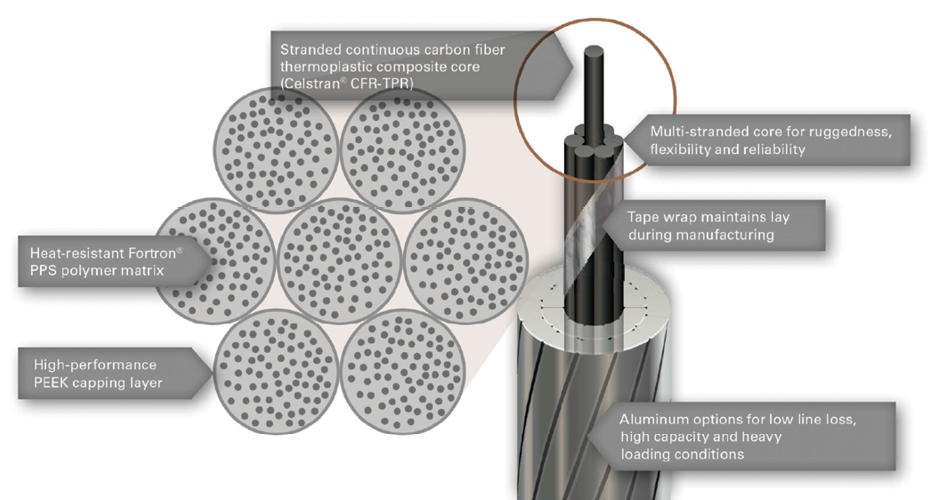
Composites are helping electrical utilities increase system capacity without the time and cost of building new conductor lines. Southwire Co. and Celanese have jointly developed a new type of high performance high-temperature low-sag conductor called the C7™ Overhead Conductor. The multi-strand thermoplastic-composite core combines carbon fiber-reinforced PPS with a PEEK capping layer, providing very-high resistance to chemicals, heat, galvanic corrosion, and abrasion. (Image courtesy of Southwire Co., LLC.)
Concrete has very-high compression strength but poor tensile strength, which is why it’s so often reinforced with rebar. In the case of below-grade foundation walls, concrete’s brittleness tends to make it crack under the constant pressure of soil backfill within a few years of installation, leading to leaky basements. Further, because concrete is porous and a poor thermal insulator, moisture and cold can easily pass through walls, making below-ground spaces damp and cool (the perfect breeding ground for mold and mildew) unless further improvements are made.
Composites, in contrast, can be engineered for both high tensile and compressive strength, plus are inherently moisture and corrosion resistant, making them interesting materials for foundation walls. Since composites are naturally thermally insulating and that property can be improved via polymer foams, composite foundation walls can be insulating, helping transform cold, damp, smelly basements into warm, dry spaces and creating an airtight transition between the foundation and upper floors.
The Epitome foundation-wall system consists of foam-cored fiberglass panels using Ashland’s Modar fire-retardant, non-halogenated modified (thermoset) acrylic polymer and produced in a proprietary process. Panels are 18 cm thick, 7.4 m long, and 2.8 m tall and carry an R-16.5 insulation value. Thermal insulation (provided by urethane foam) and vapor barrier are integral to the panels, allowing them to last the life of the foundation. Pultruded flanges attached to panel ends facilitate attachment to adjacent panels. Panels also feature integrally molded nominally sized studs (41 cm on center) and stud cavities, which act as pilasters and make it easy for contractors to install mechanicals or additional insulation (boosting R-values to 30+).
Unlike poured-concrete foundation walls, which can take up to two weeks to cure depending on weather, the new system’s custom-fabricated panels arrive in a single delivery ready to install. On a standard-size dwelling, foundation walls can be erected in less than two hours by crews with standard trade skills and minimal training. This not only gives builders more control (reducing weather and subcontractor constraints) but also means contractors can install more homes per season with the same crew, and that a given home can be made weather-tight much faster.
The structurally robust composite panels are designed to withstand six-times greater pressures than a standard sand backfill load, and each 7.4 m panel can withstand 272 tonnes of down force, resulting in a maximum allowable house load of 13,245 kg/linear meter. Usable on any soil suitable for backfilling, this wall system is said to be cost-competitive anywhere concrete foundations are installed.
Consumers benefit too with warmer, drier, more energy-efficient foundations that are structurally dependable for the life of the home. The sanitizable panels are highly fire retardant (having passed demanding U.S. National Fire Protection Association 286 room corner burn testing), so no thermal barriers like gypsum drywall are needed before occupancy. This lets homeowners finish basements at their convenience. Since panels are 18-cm deep, not 31-cm deep like most insulated, stud-framed cast foundation walls, the system actually offers more usable living space inside the basement―adding an average of 9.3 m2(roughly the size of a small bedroom). Finally, panel construction and installation use fewer natural resources and less energy for a smaller carbon footprint than concrete.
The new panel system has met strict guidelines for in-plant quality and has successfully completed rigorous testing and building code requirements set by NTA Inc., accredited by the International Accreditation Service (IAS), a subsidiary of the International Code Council (ICC), which grants Epitome foundation walls national code compliance for residential foundations. The technology also won several awards for industry-changing technology at the Composites and Advanced Materials Exposition (CAMX) last fall.
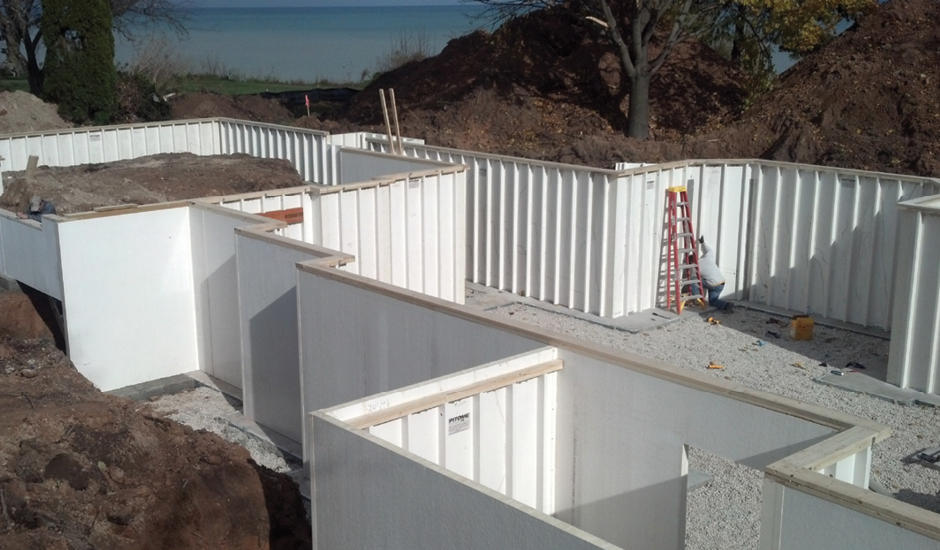
A new foundation-wall system of foam-cored fiberglass panels replaces poured concrete to create warmer, drier, more energy-efficient foundations that reportedly are structurally dependable for the life of the home. On a standard-size dwelling, foundation walls can be erected in less than two hours by crews with standard trade skills and minimal training. The wall system is said to be cost competitive anywhere concrete foundations are installed. (Photo courtesy of Composite Panel Systems, LLC.)
Acknowledgements
Information in this article was largely drawn from interviews with Claude Gaumond, Groupe Médical Gaumond, and Claude Baril, Composites Atlantic Ltd.; Habib Dagher, University of Maine-Orono; Ted Harris and Dustin Troutman, Creative Pultrusions, Inc., and David W. Johnson, Ebert Composites Corp.; Michael Ruby, Celanese Corp., and Mark Lancaster, Southwire Company, LLC; and Thom Johnson, Ashland, Inc.
Reinforced Plastics Move into Non-Traditional Markets
Composites improve performance, reduce mass and costs, increase design versatility, and extend use life
Previous Article Next Article
By Peggy Malnati
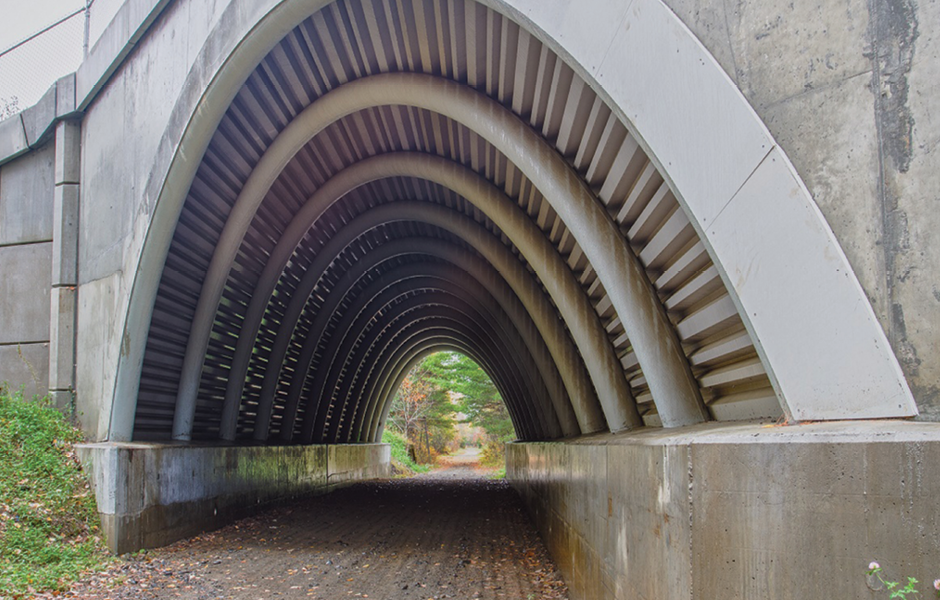
Composites are helping speed up bridge construction (see the second section of this article).
Composites have a long history of use in industries such as automotive, aviation/aerospace, and sporting goods, but their use is now expanding into less-traditional markets like medical, infrastructure, power transmission, and building & construction, where they are displacing metals, wood and wood products, and even concrete. Composites are moving into non-traditional segments for a variety of reasons: they improve part performance, reduce mass and costs, increase design flexibility through parts consolidation, reduce or eliminate secondary operations, don’t corrode or rot, extend durability and use life, increase production speeds, and produce more consistent products. Some interesting examples of recent applications in non-traditional markets are below.
Hyperbaric Oxygen Therapy Chambers
Hyperbaric (above atmospheric pressure) oxygen therapy (HBOT) is best known for saving divers with “the bends” (decompression sickness), but it’s also a well-documented medical treatment for many chronic and acute conditions (i.e., altitude sickness, severe anemia, brain abscesses, carbon-monoxide poisoning, arterial embolism, radiation injuries, and sudden vision or hearing loss). Because HBOT temporarily enables blood to carry more oxygen, it also helps fight infection, reduces inflammation, and speeds healing in cases of crushing injuries, skin/bone infections, skin grafts, and burns.
Traditionally HBOT sessions were administered in rigid single- or multi-person chambers capable of pressures up to 3 ATA (atmosphere absolute, i.e., pressure above normal atmospheric pressure). These heavy glass and metal units were permanent installations that were costly to install and maintain, so treatment costs were high and chambers were only available at large hospitals or naval/diving and recompression centers.
More recently, single-person, soft-sided portable systems capable of pressures to 1.4 ATA were introduced. These polymer-coated fabric chambers don’t provide the same efficacy as the higher-pressure rigid systems, but they are less costly to purchase and maintain, making them affordable for small clinics and individuals. They also weigh far less so are portable/transportable and can be stowed until needed.
Now, a new composites-intensive hyperbaric chamber bridges the gap between costly/high-pressure units and portable units operating at less than half the pressures. The HematoCaresystemfrom Groupe Médical Gaumond (GMG; Montréal, Quebec, Canada) took a decade to develop, and later this year will become the first HBOT system to achieve global agency approvals. Working with National Research Council Canada’s Industrial Research Assistance Program (IRAP; Ottawa, Ontario, Canada), Cytec Industries Inc. (Woodland Park, New Jersey, USA), and Composites Atlantic Ltd. (Lunenburg, Nova Scotia, Canada), the team’s goal was to develop a lightweight chamber capable of maintaining 3 ATA without leaking or tearing, but still affordable for small clinics and individuals. (Certification at 3 ATA operating pressures means chambers are tested at pressures six-times higher, so mechanical requirements are demanding and flame-retardancy standards stringent, owing to oxygen’s combustibility.)
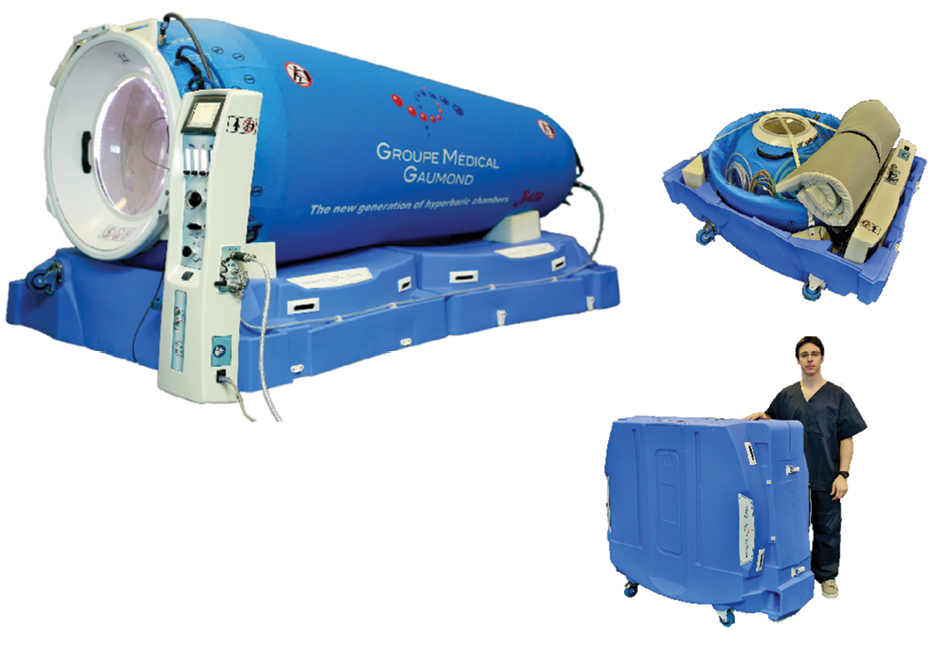
Hyperbaric oxygen therapy (HBOT) is a medical treatment for a variety of chronic and acute conditions. A new type of composites-intensive hyperbaric chamber has a cone-shaped hollow chamber that’s filament wound using high-performance aramid fiber impregnated with urethane. Rigid composite end caps (infused with gel-coated, fiberglass-reinforced epoxy and fitted with curved acrylic windows) are held snuggly in place on either end of the flexible chamber by pressure alone and provide patient egress. All standard system components pack into a tough two-piece HDPE rotomolded case with casters (insets). (Photos courtesy of Groupe Médical Gaumond.)
Years of testing and evaluation led to development of a filament-wound cone-shaped hollow chamber produced by Composites Atlantic using high-performance aramid fiber from Teijin Ltd. (Osaka, Japan) impregnated with custom-formulated urethane.
Rather than make ill patients enter through a double-zippered opening at the top, like with most soft-sided units, GMG eases patient access by creating door- and window-equipped rigid composite end caps that fit snuggly into the flexible chamber yet aren’t permanently attached. GMG infuses the parts in gel-coated, fiberglass-reinforced epoxy. After post-mold trimming, an aluminum support ring is bonded into each end cap followed by insertion of a rubber O-ring, a curved acrylic window, and a final aluminum locking ring. Clever design inside the end caps allows optional rails to be added to support patients entering on stretchers.
All standard system components pack into a tough, two-piece case with casters that’s rotomolded from unreinforced high-density polyethylene. When the chamber is deployed, carrying-case halves open to form a base that supports the chamber, while foam blocks prevent it from rolling. With experience, two people can deploy the system in 15 minutes and repack it in half that time. The system is warranted for ten years/4,000 cycles and, with a 125-kg packed weight, is said to be the lightest, least-expensive HBOT chamber certified to 3 ATA working pressures, making it adaptable for home, clinic, or hospital use.
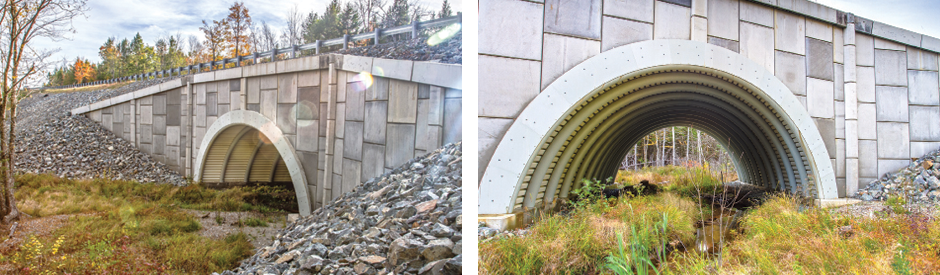
A composites-intensive bridge technology developed by the University of Maine is said to provide a better and faster way to replace a wide variety of concrete and other bridges. The composite bridge uses “inflatable arches”―Portland cement-filled resin-infused textile sleeves that provide a permanent form for concrete—creating an exoskeleton that eliminates the need for rebar and protects concrete from water and environmental damage. (Photos courtesy of University of Maine.)
Composites for Rapid Bridge Replacement
With increasingly unpredictable weather, natural disasters, and civil unrest plaguing many regions, it’s increasingly important to be able to replace damaged or destroyed bridges rapidly. Even in settled areas, aging infrastructure on bridges that are over their limit and beyond their service life means local and regional governments need ways to replace bridges quickly and cost effectively, preferably with materials offering longer use life.
Traditional precast and cast-in-place concrete bridges have issues. Precast components are very heavy and costly to move, while extensive formwork must be built before casting-in-place, followed by long waits as the concrete cures, adding weeks or months to jobs, particularly in cold and wet conditions. Although fully cured concrete is durable—withstanding broad temperature fluctuations, high winds, heavy loads, and abrasion—it can be affected by cold-weather salt deicers used on roads and bridges (causing concrete spalling and rebar corrosion) and water intrusion (necessitating installation and maintenance of expansion joints to prevent freezing water from splitting components). Both situations eventually can compromise bridge integrity.
Additionally, concrete’s high specific gravity adds significant “dead weight,” reducing a bridge’s true carrying capacity. The shear mass of precast components and the high labor requirements of cast concrete require the use of heavy equipment and large work crews. This increase risks for negative environmental impacts and often requires environmental-impact studies be conducted prior to permitting, slowing projects and adding cost. Furthermore, shutting down busy bridges for repair/rebuilding increases traffic delays and commute times, frustrates commuters, and can harm local commerce, all of which mean municipalities want bridges fixed and back in service as soon as possible.
A composites-intensive bridge technology developed by University of Maine’s Advanced Structures & Composites Center and commercialized by Advanced Infrastructure Technologies (both in Orono, Maine, USA) is said to provide a better, faster way to replace a wide variety of concrete bridges, freeway underpasses/overpasses, and railroad bridges. The project began with three ambitious goals: replace concrete formwork and rebar, use efficient arched structures, and produce components at the worksite.
Researchers reviewed and rejected many design options before deciding to produce formworks using inflatable fabric sleeves. Ultimately a proprietary fabric blending glass, carbon, and other fibers and infused with epoxy-vinyl ester resin (selected for its durability, ductility, and favorable adhesion to concrete) was developed. In use, the custom-designed sleeves are cut to length and width, joined to form a tube, and mounted to custom fixtures. Tube ends are then sealed and sleeves are inflated with air prior to resin impregnation. Cure occurs at room temperature and normal pressures.

A pultruded 3-D composite technology called Transonite developed by Ebert Composites Corp. is used for a wide variety of lightweight panels, providing reinforcement in all three orthogonal directions. A variety of fibers, fabrics, and thermoset or thermoplastic resins can be used, making it easy to tailor performance, cost, and mass to meet application needs. An internal “wave” pattern of reinforcement is said to withstand deflection and high shear forces. (Photo courtesy of Creative Pultrusions, Inc.)
Arches are then moved to a job site, positioned, and concrete footings are poured. Next, holes are cut at the top of each arch and a proprietary grade of Portland cement (which is slightly expansive and bonds well to the composite sleeve) is poured into each arch and allowed to cure. Then decking (conventional or composite) is installed over the arches, sand backfill is used, and a final wear surface (asphalt or polymer concrete) is applied.
Functionally, inflatable arches provide a permanent form for concrete, create an exoskeleton that eliminates the need for rebar, and protect concrete from water intrusion and other environmental damage. Installed costs can be 20% lower than conventional materials, yet these joint- and rebar-free bridges are designed to last at least twice the typical 50- to 70-year lifespan of conventional bridges. Eliminating expansion joints and rebar means the new technology is less prone to fatigue, corrosion, and water infiltration, reducing maintenance costs and time. Smart sensors can be installed for active bridge monitoring. A small bridge can be completely replaced in as little as one week, versus months with conventional construction.
Durable 3-D Composite Panel Structures
A unique pultruded 3-D composite developed by Ebert Composites Corp. (Chula Vista, Califorinia, USA) and licensed by Creative Pultrusions, Inc. (Alum Bank, Pennsylvania, USA) provides x, y, and z-axis reinforcement (structural fibers in all three orthogonal directions). This increases panel durability by greatly improving peel strength and delamination resistance and boosting torsional rigidity. Other benefits include extremely high strength/weight ratios, thermal and acoustical insulation, and elimination of corrosion.
Called Transonite, the technology (now in its third generation) is covered under nine U.S. patents and is produced on unique equipment via an automated process (both developed by Ebert) capable of supporting high-volume manufacturing at low costs. Functioning like I-beams, z-axis fibers are precut rovings (e.g., glass, carbon, aramid, basalt) that are pushed through top and bottom skins with or without a core in place. The “clinched/riveted” rovings in one discrete fiber bundle aren’t connected to fibers in adjacent bundles (fibers are not stitched together), yet the unbroken fibers form regular “columns” in the through-thickness direction. Cores may be solid or foamed resins or even balsa wood.
Special tooling keeps skins and fiber columns from collapsing prior to infusion/impregnation, which ties fibers, cores, and skins. Additional skin layers are added to lock rovings into original skins and improve surface finish. Finally, the pultruded sandwich is pulled through a heated die to catalyze and cure the resin, which typically is a thermoset (unsaturated polyester, vinyl ester, or polyurethane) but also can be thermoplastic.
Thanks to process versatility, a wide variety of fibers, fabrics, and resins can be used, making it easy to tailor performance, cost, and mass to meet application needs. Performance can be changed by manipulating panel thickness (13-102 mm), skin thickness (1.27-10.2 mm), skin/core ratio, panel width (15-259 cm), and density of z-axis fibers (0.08-2.48/cm2). Since it’s a continuous process, panels can be cut to nearly any length greater than 2.5 cm, yielding large, clean surfaces free of seams, which is important in situations where moisture intrusion is a concern and/or aesthetics are important.
The angle of z-axis fiber placement is another interesting variable. While 90° degrees to the skins is most common, recent developments permit addition of very-high-shear layers of fibers positioned nearly 45° to the z-axis prior to resin impregnation, creating an internal “wave” pattern that withstands deflection and high shear forces.
Post-mold trimming is done via waterjet or diamond tools. Bare panels can be finished with a variety of surfaces for interior or exterior use, including carpet, antiskid aggregate, wallpaper, and polyurea.
Transonite technology is currently used for floors and sidewalls on recreational vehicles and truck trailers and was demonstrated on a small building in a Chinese solar competition. Other uses include panels for ballistic protection, air-cargo containers, marine uses, furniture, rails, bridge decks, industrial mats, and automotive uses.
Composites for Power Transmission
In an industry long dominated by metals, uses of composites in high-voltage overhead electrical conductors are increasing power-transmission capabilities and capacities. As populations increase and nations consume more power, much of the planet’s electrical-transmission infrastructure is outdated and exceeding its capacity limits. Load growth aside, utilities in many countries legally are required to connect to increasing amounts of solar and wind energy generated by public and private sources, which expands and further stresses aging electrical infrastructure.
To meet projected demand and accommodate alternative power generation, electric utilities have three ways to increase system capacity:
- build new lines (a costly approach requiring difficult-to-secure public consent and rights-of-way for land, and even longer waits for permitting and construction);
- “reconductoring” (restringing) existing lines with conductor of the same type (which may necessitate strengthening or replacing existing towers and poles, and still involves waits for permitting and construction); or
- reconductoring existing lines with more efficient conductor (the fastest and least costly approach).
Overhead transmission lines must meet many challenging standards, including minimum clearance to ground, ice and wind loads, and thermal-operating limits to prevent damage. Lines are designed to maintain a safe distance from the ground at a rated electrical load under specific environmental conditions. Line sag and therefore ground clearance are affected by both thermal and mechanical issues.
When lines are strung over areas with mountains, bodies of water, or prone to severe weather, higher performance high-temperature low-sag (HTLS) conductor is typically required instead of conventional aluminum conductor steel-reinforced (ACSR) or other alloy-based products. Conductors using lighter, stronger core materials with broader thermal performance can transmit more power during both normal and contingency operations while maintaining required line clearance.
Recent developments in high-strength, low-sag lightweight composite cores to replace stranded steel cores have changed how transmission lines are designed. Carbon fiber-reinforced epoxy cores typically use a single (monolithic) rod rather than multiple strands traditionally used with steel cores to eliminate a single point of failure. Monolithic cores require a larger bending radius than traditional stranded cores and must be handled carefully by trained technicians using special connectors and terminations.
Another composite product features aluminum metal-matrix composites (MMCs, aluminum oxide fibers in a high-purity aluminum matrix). The multi-strand MMC products use traditional fittings but also have a higher bend radius than traditional ACSR conductor. They are rated for high operating temperatures (to 240°C). However, their high cost means they are typically used for only the most restrictive installation environments.
Given the limitations of current HTLS composite conductors, Southwire Company, LLC (Carrollton, Georgia, USA, one of the world’s largest wire and cable producers), and Celanese Corp. (Dallas, Texas, USA) have jointly developed a new type of HTLS product called C7™ Overhead Conductor. The multi-strand pultruded thermoplastic-composite core combines carbon fiber-reinforced polyphenylene sulfide (PPS) with a polyetheretherketone (PEEK) capping layer for very-high resistance to chemicals, heat, galvanic corrosion, and abrasion.
The new conductor carries approximately twice the current as conventional ASCR conductor and provides a high continuous-use (180°C) and even higher emergency-use (225°C) rating, helping utilities maximize energy throughput. The installation-friendly conductor provides greater flexibility and bending radius than monolithic thermoset and MMC composites and works with conventional fittings, reducing crew training time and increasing familiarity. A multi-strand core means greater reliability and redundancy during installation and long-term use compared with monolithic-core conductor.
The new product combines high performance, excellent value, and the toughness and durability to deliver at least a 40-year service-life expectancy, helping utilities increase capacity without the cost of installing new infrastructure. By reducing power losses, transmission is more efficient, and utilities deliver more billable power to customers while avoiding infrastructure upgrades, which also benefit power consumers and reduce environmental impact of the power-transmission grid.
Composite Foundation Walls for Homes
A new composite-based foundation-wall system introduced last year by Composite Panel Systems, LLC (Eagle River, Wisconsin, USA) with support from panel fabricator Fiber-Tech Industries, Inc. (Spokane, Washington, USA) and resin supplier Ashland Performance Materials (a commercial unit of Ashland Inc., Dublin, Ohio, USA) is set to overturn the century-old trend of using poured concrete to form vertical foundation walls on residential homes, at comparable costs, faster installation, and far-better performance.
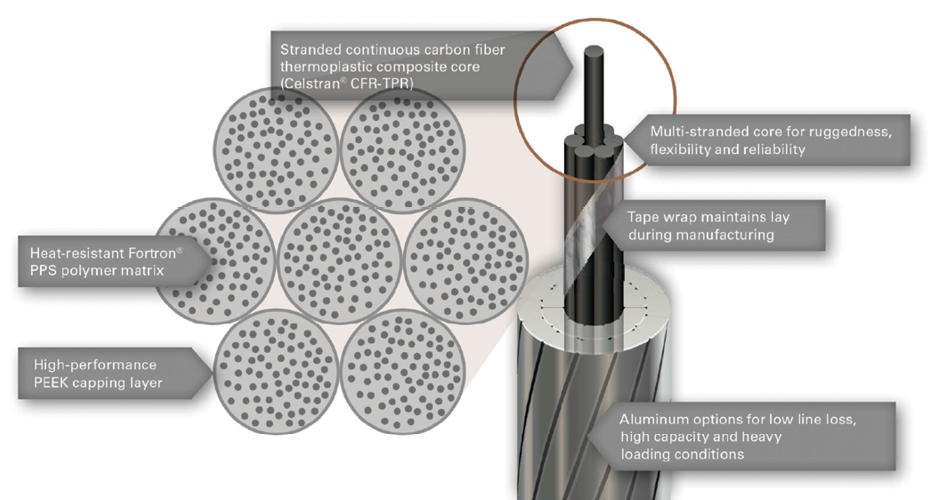
Composites are helping electrical utilities increase system capacity without the time and cost of building new conductor lines. Southwire Co. and Celanese have jointly developed a new type of high performance high-temperature low-sag conductor called the C7™ Overhead Conductor. The multi-strand thermoplastic-composite core combines carbon fiber-reinforced PPS with a PEEK capping layer, providing very-high resistance to chemicals, heat, galvanic corrosion, and abrasion. (Image courtesy of Southwire Co., LLC.)
Concrete has very-high compression strength but poor tensile strength, which is why it’s so often reinforced with rebar. In the case of below-grade foundation walls, concrete’s brittleness tends to make it crack under the constant pressure of soil backfill within a few years of installation, leading to leaky basements. Further, because concrete is porous and a poor thermal insulator, moisture and cold can easily pass through walls, making below-ground spaces damp and cool (the perfect breeding ground for mold and mildew) unless further improvements are made.
Composites, in contrast, can be engineered for both high tensile and compressive strength, plus are inherently moisture and corrosion resistant, making them interesting materials for foundation walls. Since composites are naturally thermally insulating and that property can be improved via polymer foams, composite foundation walls can be insulating, helping transform cold, damp, smelly basements into warm, dry spaces and creating an airtight transition between the foundation and upper floors.
The Epitome foundation-wall system consists of foam-cored fiberglass panels using Ashland’s Modar fire-retardant, non-halogenated modified (thermoset) acrylic polymer and produced in a proprietary process. Panels are 18 cm thick, 7.4 m long, and 2.8 m tall and carry an R-16.5 insulation value. Thermal insulation (provided by urethane foam) and vapor barrier are integral to the panels, allowing them to last the life of the foundation. Pultruded flanges attached to panel ends facilitate attachment to adjacent panels. Panels also feature integrally molded nominally sized studs (41 cm on center) and stud cavities, which act as pilasters and make it easy for contractors to install mechanicals or additional insulation (boosting R-values to 30+).
Unlike poured-concrete foundation walls, which can take up to two weeks to cure depending on weather, the new system’s custom-fabricated panels arrive in a single delivery ready to install. On a standard-size dwelling, foundation walls can be erected in less than two hours by crews with standard trade skills and minimal training. This not only gives builders more control (reducing weather and subcontractor constraints) but also means contractors can install more homes per season with the same crew, and that a given home can be made weather-tight much faster.
The structurally robust composite panels are designed to withstand six-times greater pressures than a standard sand backfill load, and each 7.4 m panel can withstand 272 tonnes of down force, resulting in a maximum allowable house load of 13,245 kg/linear meter. Usable on any soil suitable for backfilling, this wall system is said to be cost-competitive anywhere concrete foundations are installed.
Consumers benefit too with warmer, drier, more energy-efficient foundations that are structurally dependable for the life of the home. The sanitizable panels are highly fire retardant (having passed demanding U.S. National Fire Protection Association 286 room corner burn testing), so no thermal barriers like gypsum drywall are needed before occupancy. This lets homeowners finish basements at their convenience. Since panels are 18-cm deep, not 31-cm deep like most insulated, stud-framed cast foundation walls, the system actually offers more usable living space inside the basement―adding an average of 9.3 m2(roughly the size of a small bedroom). Finally, panel construction and installation use fewer natural resources and less energy for a smaller carbon footprint than concrete.
The new panel system has met strict guidelines for in-plant quality and has successfully completed rigorous testing and building code requirements set by NTA Inc., accredited by the International Accreditation Service (IAS), a subsidiary of the International Code Council (ICC), which grants Epitome foundation walls national code compliance for residential foundations. The technology also won several awards for industry-changing technology at the Composites and Advanced Materials Exposition (CAMX) last fall.
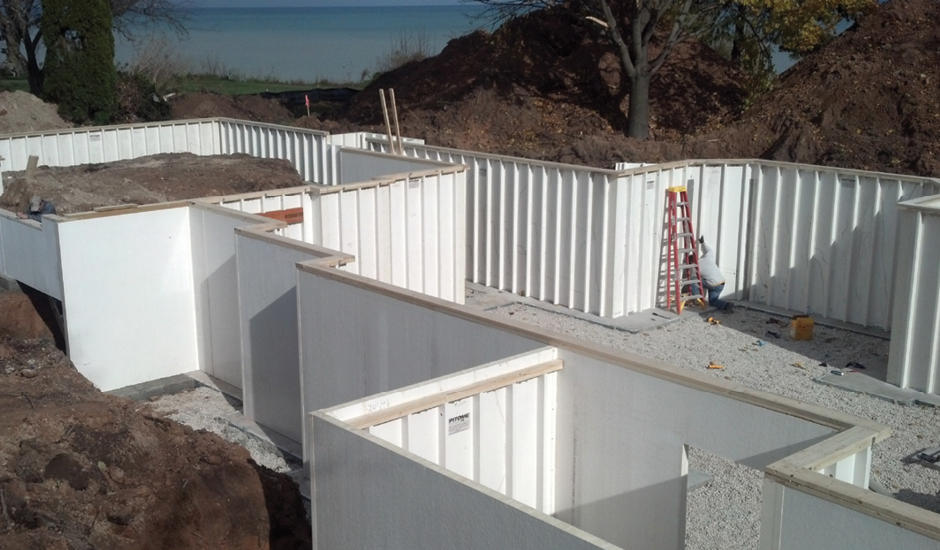
A new foundation-wall system of foam-cored fiberglass panels replaces poured concrete to create warmer, drier, more energy-efficient foundations that reportedly are structurally dependable for the life of the home. On a standard-size dwelling, foundation walls can be erected in less than two hours by crews with standard trade skills and minimal training. The wall system is said to be cost competitive anywhere concrete foundations are installed. (Photo courtesy of Composite Panel Systems, LLC.)
Acknowledgements
Information in this article was largely drawn from interviews with Claude Gaumond, Groupe Médical Gaumond, and Claude Baril, Composites Atlantic Ltd.; Habib Dagher, University of Maine-Orono; Ted Harris and Dustin Troutman, Creative Pultrusions, Inc., and David W. Johnson, Ebert Composites Corp.; Michael Ruby, Celanese Corp., and Mark Lancaster, Southwire Company, LLC; and Thom Johnson, Ashland, Inc.
Reinforced Plastics Move into Non-Traditional Markets
Composites improve performance, reduce mass and costs, increase design versatility, and extend use life
Previous Article Next Article
By Peggy Malnati
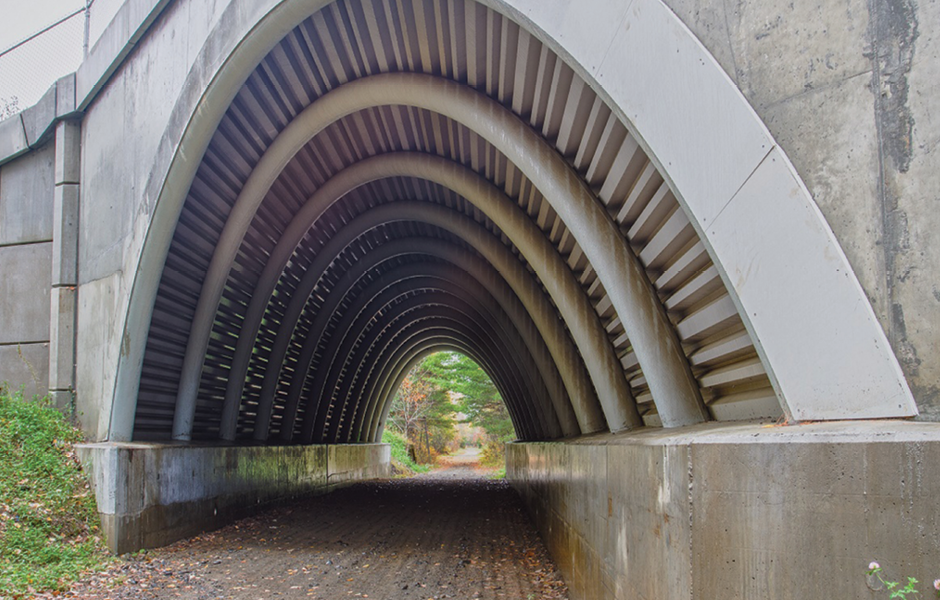
Composites are helping speed up bridge construction (see the second section of this article).
Composites have a long history of use in industries such as automotive, aviation/aerospace, and sporting goods, but their use is now expanding into less-traditional markets like medical, infrastructure, power transmission, and building & construction, where they are displacing metals, wood and wood products, and even concrete. Composites are moving into non-traditional segments for a variety of reasons: they improve part performance, reduce mass and costs, increase design flexibility through parts consolidation, reduce or eliminate secondary operations, don’t corrode or rot, extend durability and use life, increase production speeds, and produce more consistent products. Some interesting examples of recent applications in non-traditional markets are below.
Hyperbaric Oxygen Therapy Chambers
Hyperbaric (above atmospheric pressure) oxygen therapy (HBOT) is best known for saving divers with “the bends” (decompression sickness), but it’s also a well-documented medical treatment for many chronic and acute conditions (i.e., altitude sickness, severe anemia, brain abscesses, carbon-monoxide poisoning, arterial embolism, radiation injuries, and sudden vision or hearing loss). Because HBOT temporarily enables blood to carry more oxygen, it also helps fight infection, reduces inflammation, and speeds healing in cases of crushing injuries, skin/bone infections, skin grafts, and burns.
Traditionally HBOT sessions were administered in rigid single- or multi-person chambers capable of pressures up to 3 ATA (atmosphere absolute, i.e., pressure above normal atmospheric pressure). These heavy glass and metal units were permanent installations that were costly to install and maintain, so treatment costs were high and chambers were only available at large hospitals or naval/diving and recompression centers.
More recently, single-person, soft-sided portable systems capable of pressures to 1.4 ATA were introduced. These polymer-coated fabric chambers don’t provide the same efficacy as the higher-pressure rigid systems, but they are less costly to purchase and maintain, making them affordable for small clinics and individuals. They also weigh far less so are portable/transportable and can be stowed until needed.
Now, a new composites-intensive hyperbaric chamber bridges the gap between costly/high-pressure units and portable units operating at less than half the pressures. The HematoCaresystemfrom Groupe Médical Gaumond (GMG; Montréal, Quebec, Canada) took a decade to develop, and later this year will become the first HBOT system to achieve global agency approvals. Working with National Research Council Canada’s Industrial Research Assistance Program (IRAP; Ottawa, Ontario, Canada), Cytec Industries Inc. (Woodland Park, New Jersey, USA), and Composites Atlantic Ltd. (Lunenburg, Nova Scotia, Canada), the team’s goal was to develop a lightweight chamber capable of maintaining 3 ATA without leaking or tearing, but still affordable for small clinics and individuals. (Certification at 3 ATA operating pressures means chambers are tested at pressures six-times higher, so mechanical requirements are demanding and flame-retardancy standards stringent, owing to oxygen’s combustibility.)
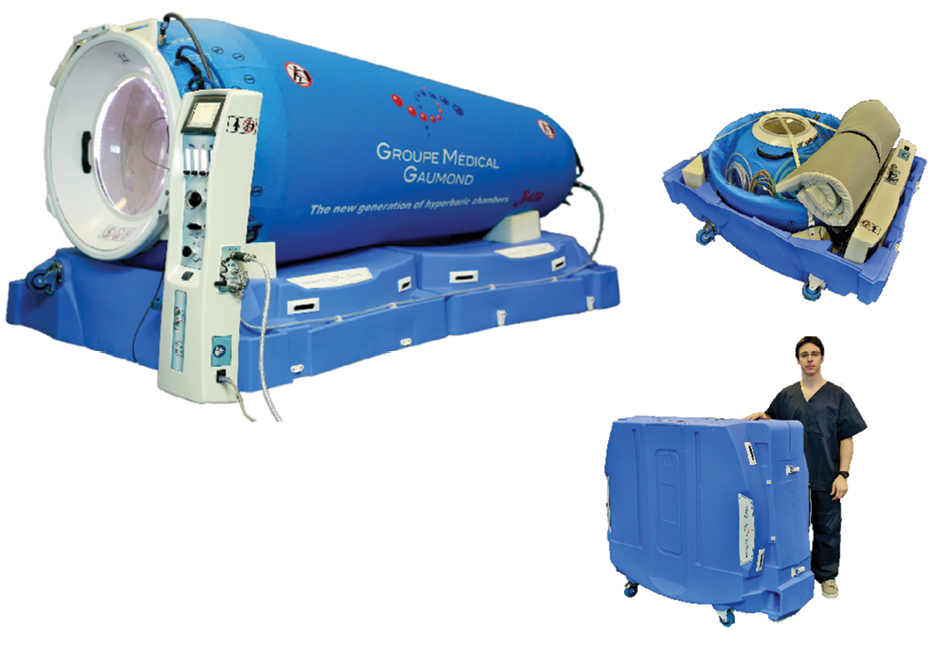
Hyperbaric oxygen therapy (HBOT) is a medical treatment for a variety of chronic and acute conditions. A new type of composites-intensive hyperbaric chamber has a cone-shaped hollow chamber that’s filament wound using high-performance aramid fiber impregnated with urethane. Rigid composite end caps (infused with gel-coated, fiberglass-reinforced epoxy and fitted with curved acrylic windows) are held snuggly in place on either end of the flexible chamber by pressure alone and provide patient egress. All standard system components pack into a tough two-piece HDPE rotomolded case with casters (insets). (Photos courtesy of Groupe Médical Gaumond.)
Years of testing and evaluation led to development of a filament-wound cone-shaped hollow chamber produced by Composites Atlantic using high-performance aramid fiber from Teijin Ltd. (Osaka, Japan) impregnated with custom-formulated urethane.
Rather than make ill patients enter through a double-zippered opening at the top, like with most soft-sided units, GMG eases patient access by creating door- and window-equipped rigid composite end caps that fit snuggly into the flexible chamber yet aren’t permanently attached. GMG infuses the parts in gel-coated, fiberglass-reinforced epoxy. After post-mold trimming, an aluminum support ring is bonded into each end cap followed by insertion of a rubber O-ring, a curved acrylic window, and a final aluminum locking ring. Clever design inside the end caps allows optional rails to be added to support patients entering on stretchers.
All standard system components pack into a tough, two-piece case with casters that’s rotomolded from unreinforced high-density polyethylene. When the chamber is deployed, carrying-case halves open to form a base that supports the chamber, while foam blocks prevent it from rolling. With experience, two people can deploy the system in 15 minutes and repack it in half that time. The system is warranted for ten years/4,000 cycles and, with a 125-kg packed weight, is said to be the lightest, least-expensive HBOT chamber certified to 3 ATA working pressures, making it adaptable for home, clinic, or hospital use.
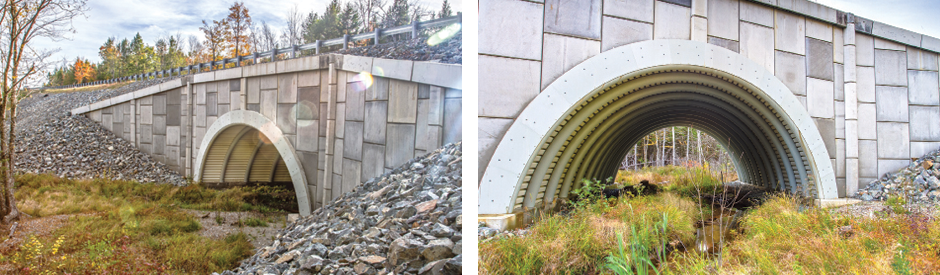
A composites-intensive bridge technology developed by the University of Maine is said to provide a better and faster way to replace a wide variety of concrete and other bridges. The composite bridge uses “inflatable arches”―Portland cement-filled resin-infused textile sleeves that provide a permanent form for concrete—creating an exoskeleton that eliminates the need for rebar and protects concrete from water and environmental damage. (Photos courtesy of University of Maine.)
Composites for Rapid Bridge Replacement
With increasingly unpredictable weather, natural disasters, and civil unrest plaguing many regions, it’s increasingly important to be able to replace damaged or destroyed bridges rapidly. Even in settled areas, aging infrastructure on bridges that are over their limit and beyond their service life means local and regional governments need ways to replace bridges quickly and cost effectively, preferably with materials offering longer use life.
Traditional precast and cast-in-place concrete bridges have issues. Precast components are very heavy and costly to move, while extensive formwork must be built before casting-in-place, followed by long waits as the concrete cures, adding weeks or months to jobs, particularly in cold and wet conditions. Although fully cured concrete is durable—withstanding broad temperature fluctuations, high winds, heavy loads, and abrasion—it can be affected by cold-weather salt deicers used on roads and bridges (causing concrete spalling and rebar corrosion) and water intrusion (necessitating installation and maintenance of expansion joints to prevent freezing water from splitting components). Both situations eventually can compromise bridge integrity.
Additionally, concrete’s high specific gravity adds significant “dead weight,” reducing a bridge’s true carrying capacity. The shear mass of precast components and the high labor requirements of cast concrete require the use of heavy equipment and large work crews. This increase risks for negative environmental impacts and often requires environmental-impact studies be conducted prior to permitting, slowing projects and adding cost. Furthermore, shutting down busy bridges for repair/rebuilding increases traffic delays and commute times, frustrates commuters, and can harm local commerce, all of which mean municipalities want bridges fixed and back in service as soon as possible.
A composites-intensive bridge technology developed by University of Maine’s Advanced Structures & Composites Center and commercialized by Advanced Infrastructure Technologies (both in Orono, Maine, USA) is said to provide a better, faster way to replace a wide variety of concrete bridges, freeway underpasses/overpasses, and railroad bridges. The project began with three ambitious goals: replace concrete formwork and rebar, use efficient arched structures, and produce components at the worksite.
Researchers reviewed and rejected many design options before deciding to produce formworks using inflatable fabric sleeves. Ultimately a proprietary fabric blending glass, carbon, and other fibers and infused with epoxy-vinyl ester resin (selected for its durability, ductility, and favorable adhesion to concrete) was developed. In use, the custom-designed sleeves are cut to length and width, joined to form a tube, and mounted to custom fixtures. Tube ends are then sealed and sleeves are inflated with air prior to resin impregnation. Cure occurs at room temperature and normal pressures.

A pultruded 3-D composite technology called Transonite developed by Ebert Composites Corp. is used for a wide variety of lightweight panels, providing reinforcement in all three orthogonal directions. A variety of fibers, fabrics, and thermoset or thermoplastic resins can be used, making it easy to tailor performance, cost, and mass to meet application needs. An internal “wave” pattern of reinforcement is said to withstand deflection and high shear forces. (Photo courtesy of Creative Pultrusions, Inc.)
Arches are then moved to a job site, positioned, and concrete footings are poured. Next, holes are cut at the top of each arch and a proprietary grade of Portland cement (which is slightly expansive and bonds well to the composite sleeve) is poured into each arch and allowed to cure. Then decking (conventional or composite) is installed over the arches, sand backfill is used, and a final wear surface (asphalt or polymer concrete) is applied.
Functionally, inflatable arches provide a permanent form for concrete, create an exoskeleton that eliminates the need for rebar, and protect concrete from water intrusion and other environmental damage. Installed costs can be 20% lower than conventional materials, yet these joint- and rebar-free bridges are designed to last at least twice the typical 50- to 70-year lifespan of conventional bridges. Eliminating expansion joints and rebar means the new technology is less prone to fatigue, corrosion, and water infiltration, reducing maintenance costs and time. Smart sensors can be installed for active bridge monitoring. A small bridge can be completely replaced in as little as one week, versus months with conventional construction.
Durable 3-D Composite Panel Structures
A unique pultruded 3-D composite developed by Ebert Composites Corp. (Chula Vista, Califorinia, USA) and licensed by Creative Pultrusions, Inc. (Alum Bank, Pennsylvania, USA) provides x, y, and z-axis reinforcement (structural fibers in all three orthogonal directions). This increases panel durability by greatly improving peel strength and delamination resistance and boosting torsional rigidity. Other benefits include extremely high strength/weight ratios, thermal and acoustical insulation, and elimination of corrosion.
Called Transonite, the technology (now in its third generation) is covered under nine U.S. patents and is produced on unique equipment via an automated process (both developed by Ebert) capable of supporting high-volume manufacturing at low costs. Functioning like I-beams, z-axis fibers are precut rovings (e.g., glass, carbon, aramid, basalt) that are pushed through top and bottom skins with or without a core in place. The “clinched/riveted” rovings in one discrete fiber bundle aren’t connected to fibers in adjacent bundles (fibers are not stitched together), yet the unbroken fibers form regular “columns” in the through-thickness direction. Cores may be solid or foamed resins or even balsa wood.
Special tooling keeps skins and fiber columns from collapsing prior to infusion/impregnation, which ties fibers, cores, and skins. Additional skin layers are added to lock rovings into original skins and improve surface finish. Finally, the pultruded sandwich is pulled through a heated die to catalyze and cure the resin, which typically is a thermoset (unsaturated polyester, vinyl ester, or polyurethane) but also can be thermoplastic.
Thanks to process versatility, a wide variety of fibers, fabrics, and resins can be used, making it easy to tailor performance, cost, and mass to meet application needs. Performance can be changed by manipulating panel thickness (13-102 mm), skin thickness (1.27-10.2 mm), skin/core ratio, panel width (15-259 cm), and density of z-axis fibers (0.08-2.48/cm2). Since it’s a continuous process, panels can be cut to nearly any length greater than 2.5 cm, yielding large, clean surfaces free of seams, which is important in situations where moisture intrusion is a concern and/or aesthetics are important.
The angle of z-axis fiber placement is another interesting variable. While 90° degrees to the skins is most common, recent developments permit addition of very-high-shear layers of fibers positioned nearly 45° to the z-axis prior to resin impregnation, creating an internal “wave” pattern that withstands deflection and high shear forces.
Post-mold trimming is done via waterjet or diamond tools. Bare panels can be finished with a variety of surfaces for interior or exterior use, including carpet, antiskid aggregate, wallpaper, and polyurea.
Transonite technology is currently used for floors and sidewalls on recreational vehicles and truck trailers and was demonstrated on a small building in a Chinese solar competition. Other uses include panels for ballistic protection, air-cargo containers, marine uses, furniture, rails, bridge decks, industrial mats, and automotive uses.
Composites for Power Transmission
In an industry long dominated by metals, uses of composites in high-voltage overhead electrical conductors are increasing power-transmission capabilities and capacities. As populations increase and nations consume more power, much of the planet’s electrical-transmission infrastructure is outdated and exceeding its capacity limits. Load growth aside, utilities in many countries legally are required to connect to increasing amounts of solar and wind energy generated by public and private sources, which expands and further stresses aging electrical infrastructure.
To meet projected demand and accommodate alternative power generation, electric utilities have three ways to increase system capacity:
- build new lines (a costly approach requiring difficult-to-secure public consent and rights-of-way for land, and even longer waits for permitting and construction);
- “reconductoring” (restringing) existing lines with conductor of the same type (which may necessitate strengthening or replacing existing towers and poles, and still involves waits for permitting and construction); or
- reconductoring existing lines with more efficient conductor (the fastest and least costly approach).
Overhead transmission lines must meet many challenging standards, including minimum clearance to ground, ice and wind loads, and thermal-operating limits to prevent damage. Lines are designed to maintain a safe distance from the ground at a rated electrical load under specific environmental conditions. Line sag and therefore ground clearance are affected by both thermal and mechanical issues.
When lines are strung over areas with mountains, bodies of water, or prone to severe weather, higher performance high-temperature low-sag (HTLS) conductor is typically required instead of conventional aluminum conductor steel-reinforced (ACSR) or other alloy-based products. Conductors using lighter, stronger core materials with broader thermal performance can transmit more power during both normal and contingency operations while maintaining required line clearance.
Recent developments in high-strength, low-sag lightweight composite cores to replace stranded steel cores have changed how transmission lines are designed. Carbon fiber-reinforced epoxy cores typically use a single (monolithic) rod rather than multiple strands traditionally used with steel cores to eliminate a single point of failure. Monolithic cores require a larger bending radius than traditional stranded cores and must be handled carefully by trained technicians using special connectors and terminations.
Another composite product features aluminum metal-matrix composites (MMCs, aluminum oxide fibers in a high-purity aluminum matrix). The multi-strand MMC products use traditional fittings but also have a higher bend radius than traditional ACSR conductor. They are rated for high operating temperatures (to 240°C). However, their high cost means they are typically used for only the most restrictive installation environments.
Given the limitations of current HTLS composite conductors, Southwire Company, LLC (Carrollton, Georgia, USA, one of the world’s largest wire and cable producers), and Celanese Corp. (Dallas, Texas, USA) have jointly developed a new type of HTLS product called C7™ Overhead Conductor. The multi-strand pultruded thermoplastic-composite core combines carbon fiber-reinforced polyphenylene sulfide (PPS) with a polyetheretherketone (PEEK) capping layer for very-high resistance to chemicals, heat, galvanic corrosion, and abrasion.
The new conductor carries approximately twice the current as conventional ASCR conductor and provides a high continuous-use (180°C) and even higher emergency-use (225°C) rating, helping utilities maximize energy throughput. The installation-friendly conductor provides greater flexibility and bending radius than monolithic thermoset and MMC composites and works with conventional fittings, reducing crew training time and increasing familiarity. A multi-strand core means greater reliability and redundancy during installation and long-term use compared with monolithic-core conductor.
The new product combines high performance, excellent value, and the toughness and durability to deliver at least a 40-year service-life expectancy, helping utilities increase capacity without the cost of installing new infrastructure. By reducing power losses, transmission is more efficient, and utilities deliver more billable power to customers while avoiding infrastructure upgrades, which also benefit power consumers and reduce environmental impact of the power-transmission grid.
Composite Foundation Walls for Homes
A new composite-based foundation-wall system introduced last year by Composite Panel Systems, LLC (Eagle River, Wisconsin, USA) with support from panel fabricator Fiber-Tech Industries, Inc. (Spokane, Washington, USA) and resin supplier Ashland Performance Materials (a commercial unit of Ashland Inc., Dublin, Ohio, USA) is set to overturn the century-old trend of using poured concrete to form vertical foundation walls on residential homes, at comparable costs, faster installation, and far-better performance.
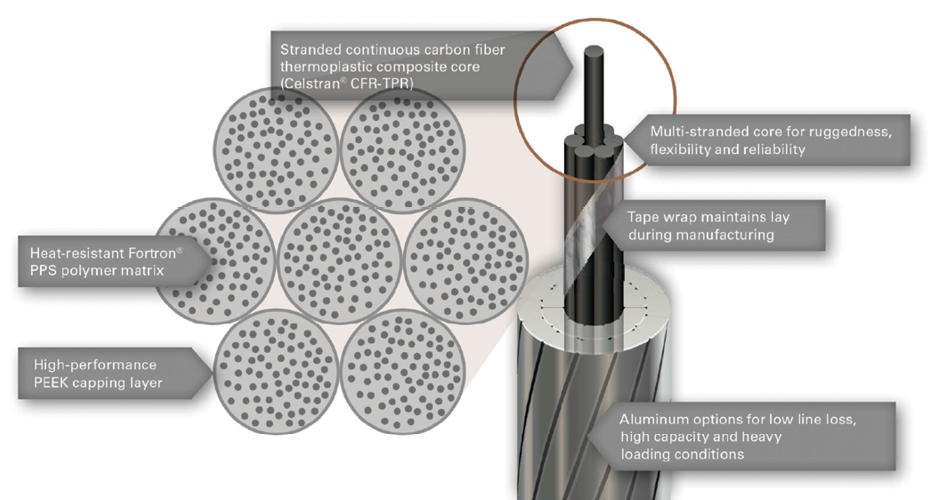
Composites are helping electrical utilities increase system capacity without the time and cost of building new conductor lines. Southwire Co. and Celanese have jointly developed a new type of high performance high-temperature low-sag conductor called the C7™ Overhead Conductor. The multi-strand thermoplastic-composite core combines carbon fiber-reinforced PPS with a PEEK capping layer, providing very-high resistance to chemicals, heat, galvanic corrosion, and abrasion. (Image courtesy of Southwire Co., LLC.)
Concrete has very-high compression strength but poor tensile strength, which is why it’s so often reinforced with rebar. In the case of below-grade foundation walls, concrete’s brittleness tends to make it crack under the constant pressure of soil backfill within a few years of installation, leading to leaky basements. Further, because concrete is porous and a poor thermal insulator, moisture and cold can easily pass through walls, making below-ground spaces damp and cool (the perfect breeding ground for mold and mildew) unless further improvements are made.
Composites, in contrast, can be engineered for both high tensile and compressive strength, plus are inherently moisture and corrosion resistant, making them interesting materials for foundation walls. Since composites are naturally thermally insulating and that property can be improved via polymer foams, composite foundation walls can be insulating, helping transform cold, damp, smelly basements into warm, dry spaces and creating an airtight transition between the foundation and upper floors.
The Epitome foundation-wall system consists of foam-cored fiberglass panels using Ashland’s Modar fire-retardant, non-halogenated modified (thermoset) acrylic polymer and produced in a proprietary process. Panels are 18 cm thick, 7.4 m long, and 2.8 m tall and carry an R-16.5 insulation value. Thermal insulation (provided by urethane foam) and vapor barrier are integral to the panels, allowing them to last the life of the foundation. Pultruded flanges attached to panel ends facilitate attachment to adjacent panels. Panels also feature integrally molded nominally sized studs (41 cm on center) and stud cavities, which act as pilasters and make it easy for contractors to install mechanicals or additional insulation (boosting R-values to 30+).
Unlike poured-concrete foundation walls, which can take up to two weeks to cure depending on weather, the new system’s custom-fabricated panels arrive in a single delivery ready to install. On a standard-size dwelling, foundation walls can be erected in less than two hours by crews with standard trade skills and minimal training. This not only gives builders more control (reducing weather and subcontractor constraints) but also means contractors can install more homes per season with the same crew, and that a given home can be made weather-tight much faster.
The structurally robust composite panels are designed to withstand six-times greater pressures than a standard sand backfill load, and each 7.4 m panel can withstand 272 tonnes of down force, resulting in a maximum allowable house load of 13,245 kg/linear meter. Usable on any soil suitable for backfilling, this wall system is said to be cost-competitive anywhere concrete foundations are installed.
Consumers benefit too with warmer, drier, more energy-efficient foundations that are structurally dependable for the life of the home. The sanitizable panels are highly fire retardant (having passed demanding U.S. National Fire Protection Association 286 room corner burn testing), so no thermal barriers like gypsum drywall are needed before occupancy. This lets homeowners finish basements at their convenience. Since panels are 18-cm deep, not 31-cm deep like most insulated, stud-framed cast foundation walls, the system actually offers more usable living space inside the basement―adding an average of 9.3 m2(roughly the size of a small bedroom). Finally, panel construction and installation use fewer natural resources and less energy for a smaller carbon footprint than concrete.
The new panel system has met strict guidelines for in-plant quality and has successfully completed rigorous testing and building code requirements set by NTA Inc., accredited by the International Accreditation Service (IAS), a subsidiary of the International Code Council (ICC), which grants Epitome foundation walls national code compliance for residential foundations. The technology also won several awards for industry-changing technology at the Composites and Advanced Materials Exposition (CAMX) last fall.
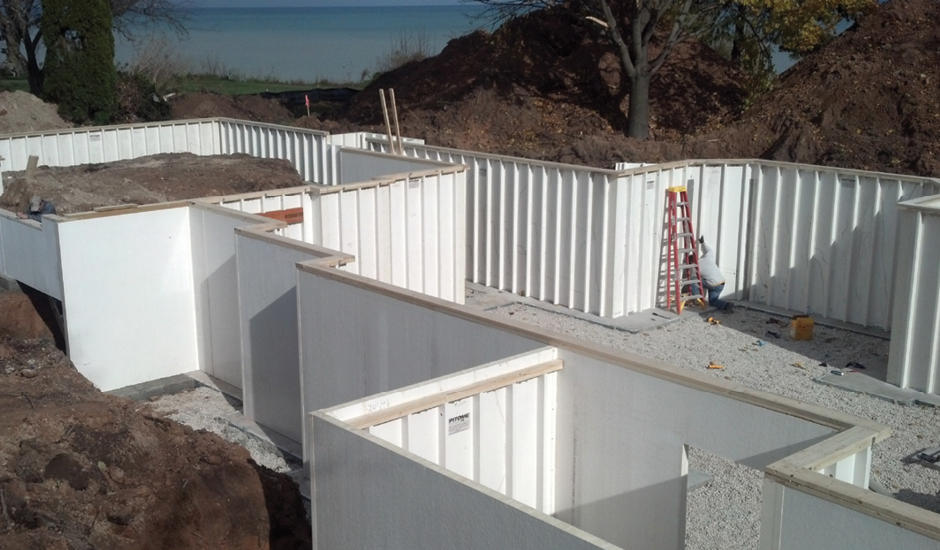
A new foundation-wall system of foam-cored fiberglass panels replaces poured concrete to create warmer, drier, more energy-efficient foundations that reportedly are structurally dependable for the life of the home. On a standard-size dwelling, foundation walls can be erected in less than two hours by crews with standard trade skills and minimal training. The wall system is said to be cost competitive anywhere concrete foundations are installed. (Photo courtesy of Composite Panel Systems, LLC.)
Acknowledgements
Information in this article was largely drawn from interviews with Claude Gaumond, Groupe Médical Gaumond, and Claude Baril, Composites Atlantic Ltd.; Habib Dagher, University of Maine-Orono; Ted Harris and Dustin Troutman, Creative Pultrusions, Inc., and David W. Johnson, Ebert Composites Corp.; Michael Ruby, Celanese Corp., and Mark Lancaster, Southwire Company, LLC; and Thom Johnson, Ashland, Inc.